Research and Thesis Projects
We continuously offer projects for suitably qualified Master and Bachelor students in the following areas: Chemical Looping Combustion, Granular Matter, Magnetic Resonance Imaging, Heterogeneous Catalysis, CO2 Capture and Conversion, Atomic Layer Deposition, …
Contact us for more details.protected page

Semester/Bachelor/Master Project
An average world temperature is steadily rising, causing the climate change. To limit the temperature increase to 1.5 °C, a target set during the Paris Agreement, not only we need to reduce the CO2 emissions, but we also need to start removing CO2. Direct Air Capture is a method to remove CO2 directly from the atmosphere using reusable sorbents. It is a quickly developing area of research, but there is still a need for better materials.
In 2023, a new class of DAC sorbents emerged, copper promoted ion exchange resin. The goal of this project is to evaluate sorbents made using different metals. The project will consist of synthesis of the materials using various metal salts, performance testing using an in-house built breakthrough rig and characterization of the produced materials. At the end, the most suitable material for DAC will be chosen.
What will you learn
During the project you will learn the essentials of Direct Air Capture, sorbent synthesis, operation of the rig simulating the industrial conditions, the industry standard characterisation techniques such as X-ray diffraction and Infrared spectroscopy, and data analysis.
If that sounds interesting to you, please contact me, so we can meet up and chat about it!
References
Chen et al., Sci. Adv, 2023, 9, eadg1956.
F. Kong, G. Rim, M. Song, C. Rosu, P. Priyadarshini, R. P. Lively, M. J. Realff and C. W. Jones, Korean Journal of Chemical Engineering, 2022, 39, 1-19.
Contact
Dominik Richert
LEE P230

Chemical Looping (CL) describes the separation of a general reaction into two or more spatially and/or temporally separated sub-reactions, by a solid intermediate[1, 2]. The solid intermediate is termed the ‘oxygen carrier’ (OC), generally a metal oxide, and is capable of releasing a significant proportion of its lattice oxygen to the gas phase in a reducing or inert environment (low pO2). The oxygen release is at the origin of the formation of oxygen vacancies, provided it is not significant enough to cause a crystal phase change (e.g. in the presence of highly reducing gases such as H2). Oxygen vacancies can be replenished by absorbing oxygen in an oxidising environment (high pO2). These sub-reactions form the basis of a ‘redox loop’.
Efficient OCs should maintain a high mechanical strength and redox stability over large numbers of cycles; they require a high oxygen storage capacity (OSC) to maximise the throughput per cycle and minimise the need for purge steps between cycles. Phase-pure perovskites (of the form ABO3) and spinels (of the form AB2O4) have been shown to have significant OSC (> 1 wt.%20). However, the oxygen uptake and release kinetics of pure perovskites are often slow. Doping either A- or B-sites with transition metals can significantly enhance these kinetics and alter the OSC[3]. In the presence of acidic gases such as CO2, basic surface oxygens are sensitive to reaction, forming surface species [4]. These block the active sites of the material and may significantly impact the oxygen uptake and release kinetics.
The work for this thesis will involve significant laboratory-based work (synthesis, material characterization) and data analysis (for which the use of MATLAB is preferred). Remote work is not possible.
The principal goals of this thesis are:
a. To detect, identify and quantify the surface species of doped perovskites as OCs for CL under relevant chemical looping conditions.
b. To correlate the oxygen uptake and release kinetics of these OCs to the presence of surface species.
Methodology:
The successful synthesis of doped perovskites will be verified by X-ray diffraction. The surface species of doped perovskites will be probed by Raman spectroscopy. Thermogravimetric analysis (TGA) will be conducted to evaluate the evolution in mass of the material subject to changes to the gas environment and temperature. TGA is also used to evaluate the oxygen release and uptake kinetics, which are ultimately correlated to the concentration and nature of surface species.
Learning Opportunities:
- To gain an understanding Chemical Looping processes and oxygen carrier materials
- To develop a skillset for lab-based material synthesis and characterization
- To apply your engineering know-how to data analysis and interpretation.
If you are interested, please get in touch!
References:
1. Oing, A., et al., Material Engineering Solutions toward Selective Redox Catalysts for Chemical-Looping-Based Olefin Production Schemes: A Review. Energy & Fuels, 2024. https://doi.org/10.1021/acs.energyfuels.4c03196
2. Zhu, X., et al., Chemical looping beyond combustion – a perspective. Energy & Environmental Science, 2020. 13(3): p. 772-804. http://dx.doi.org/10.1039/C9EE03793D
3. Li, Y., et al., Perovskites as oxygen storage materials for chemical looping partial oxidation and reforming of methane. Physical Chemistry Chemical Physics, 2024. 26(3): p. 1516-1540. http://dx.doi.org/10.1039/D3CP04626E
4. Wang, H. and W.F. Schneider, Nature and role of surface carbonates and bicarbonates in CO oxidation over RuO2. Physical Chemistry Chemical Physics, 2010. 12(24): p. 6367-6374. http://dx.doi.org/10.1039/C001683G
Contact:
Elena von Müller
LEE P230

Over the past century, atmospheric CO2 levels have steadily risen, driven primarily by the growing reliance on fossil fuels. The increase in CO2 levels has been unequivocally correlated to global warming. [1] Strategies for CO2 capture and conversion are essential for mitigating these effects. For example, the direct hydrogenation of CO2 to methanol is a sustainable alternative to the current industrial production of methanol from synthesis gas (CO and H2) obtained via reforming of methane (present in natural gas). [2] However, challenges to develop catalysts that are highly active, selective and stable over long operation remain.
For the rational design of effective catalysts, as opposed to the inefficient trial-and-error approaches, a key task is the identification of the relationships that link a catalyst’s geometric and electronic structure to its catalytic performance. Palladium-based catalysts are promising for this reaction exhibiting superior activity and stability compared to Cu-based ones. [3] Although Pd itself cannot activate CO2 efficiently and hydrogenate it to methanol, the addition of a second metal (such as Ga, Zn or In) can lead to a considerable improvement in the catalytic activity and selectivity, possibly due to modification of the electronic and/or geometric local structure around Pd. [4] However, there is still limited knowledge about the active sites in these bimetallic Pd systems. Thus, this project aims to establish structure-performance relationships in bimetallic Pd-based catalysts for CO2 hydrogenation to methanol.
We are seeking for motivated students to join our pioneering research on CO₂ hydrogenation, contributing to solutions for combating climate change by developing a fundamental understanding of catalyst behavior. Whether you’re interested in a thesis, a semester project, or a student research assistant position, we welcome your application to be part of our team. Did this spark your interest? Then contact us!
What will you do?
- Conduct daily experiments in the chemical laboratory focused on synthesizing nanomaterials for methanol production.
- Characterize nanomaterials using advanced techniques, including X-Ray Diffraction (XRD), Pair Distribution Function (PDF) analysis, and Diffuse Reflectance Infrared Spectroscopy (DRIFTS).
- Evaluate catalytic performance for CO₂ hydrogenation processes aimed at sustainable methanol synthesis.
Learning Opportunities:
- Develop a foundational understanding of CO₂ utilization technologies.
- Gain practical experience in a chemical engineering laboratory environment.
- Engage at the intersection of chemistry, engineering, and materials science.
- Gain insight into cutting-edge research conducted at ETH Zürich
Contact details
Angelo Bellia
CLA H31
References
[1]: IPCC, 2023: Sections. In: Climate Change 2023: Synthesis Report. Contribution of Working Groups I, II and III to the Sixth Assessment Report of the Intergovernmental Panel on Climate Change [Core Writing Team, H. Lee and J. Romero (eds.)]. IPCC, Geneva, Switzerland, pp. 35-115, doi: 10.59327/IPCC/AR6-9789291691647
[2]: Olah, G. A., Beyond Oil and Gas: The Methanol Economy. Angew. Chem. Int. Ed. 2005, 44 (18), 2636-2639.
[3]: Jiang, X.; Nie, X.; Guo, X.; Song, C.; Chen, J. G., Recent Advances in Carbon Dioxide Hydrogenation to Methanol via Heterogeneous Catalysis. Chem. Rev. 2020, 120 (15), 7984-8034.
[4]: Ojelade, O. A.; Zaman, S. F., A Review on Pd Based Catalysts for CO2 Hydrogenation to Methanol: In-Depth Activity and DRIFTS Mechanistic Study. Catal. Surv. Asia 2020, 24 (1), 11-37.
Bachelor thesis / Master thesis / Semester project
Chemical looping (CL) is a concept, which involves the spatial or temporal separation of a desired reaction into sub-reactions with the help of a chemical intermediate. Generally, these chemical intermediates are metal oxides, so-called oxygen carriers (OCs), that are reduced by donating lattice oxygen to a sub-reaction, and that are subsequently re-oxidized during a regeneration step, thus forming a closed loop regarding the overall process. An upcoming, promising field of application for the concept of CL is the production of value-added chemicals, due several of CL’s advantages such as the potential of heat integration, in-situ product separation, and in-situ conversion of by-products to circumvent limitations given by thermodynamic equilibrium1.
The main challenge in designing competitive CL processes to produce value-added chemicals is the tendency of the OC to over-oxidize the carbon-based feedstock to COx, which has been linked to the mobility/diffusivity of oxygen in the metal oxide in several studies2,3. Hence, this project aims at improving our understanding of the oxygen release and mobility of doped perovskite (ABO3: A,B = metal cations) metal oxides as OCs and its implications on their selectivity as redox catalysts in chemical looping partial oxidation applications.
As an indicator for the oxygen mobility, the reducibility of the doped perovskites will be assessed by temperature-programmed reduction (TPR). In addition, electrical conductivity relaxation measurements will be carried out to explicitly determine the effect of doping on the oxygen diffusivity within the metal oxide structure. The tendency of the perovskites towards overoxidation of carbonaceous feedstock to CO2 will be evaluated by catalytic experiments in a fixed-bed reactor. To quantify and understand the structural changes caused by doping, the perovskites will be analyzed by powder X-ray diffraction (XRD). Our goal is to correlate the structural changes imposed by doping to the oxygen mobility and ultimately to the catalytic performance of perovskites for partial oxidation reactions.
Contact:
Alexander Oing
LEE P224
References:
[1] Zhu, X., et al. Energy Environ. Sci. vol. 13 772–804 (2020).
[2] Zhu, X., et al. ACS Catal 8, 8213–8236 (2018).
[3] Zheng, Y. et al., Appl. Catal. B 202, 51–63 (2017).
Bachelor thesis / Master thesis / Semester project
Anthropogenic CO2 emissions and the resulting effects of climate change remain as some of the most pressing issues today. Consequently, various carbon capture and storage (CCS) technologies have been proposed to drastically reduce the emission of CO2. The storage of CO2, however, still poses a crucial challenge. Furthermore, most bulk products in chemical industry are derived from fossil sources, which results in a considerable carbon footprint of a vast number of everyday consumables. In this regard, carbon capture and utilization (CCU) technologies offer a promising solution to resolve challenges concerning the permanent storage of CO2, by converting the greenhouse gas into value-added chemicals, which in turn, will also significantly contribute to the carbon neutrality of important chemical products [1].
Among CCU technologies, the hydrogenation of CO2 to methanol has attracted increasing academic interest, due to the versatile applications of methanol, such as its utilization as a fuel additive or its facile conversion to further value-added bulk chemicals [2].
CO2 + 3H2 → CH3OH + H2O, ∆HR,298K = -49.5 kJ/mol
The main challenge of this reaction is the activation of CO2, which requires high energies and therefore high temperatures (e.g. T > 250 °C at 20 bar), at which the competing reverse water-gas shift (RWGS) reaction becomes thermodynamically favored, converting CO2 into the undesired by-product CO [3]:
CO2 + H2 → CO + H2O, ∆HR,298K = +41.1 kJ/mol
Recently, mixed metal oxide catalysts such as ZnZrOx have been reported to catalyze the hydrogenation of CO2 at very high methanol selectivity (> 90 %) and good CO2 conversion (10 %), while showing no sign of deactivation for over 500 hours of time on stream [4].
Our group is interested in attaining a fundamental understanding of catalyzing the hydrogenation of CO2 to methanol over ZnZrOx catalysts to fill the gaps where current knowledge is still lacking. We are also looking into finding new mixed metal oxide formulations to further increase the methanol productivity of this type of catalyst. In doing so, we synthesize the catalysts via impregnation or co-precipitation routes and subsequently carry out an in-depth structural characterization. Ultimately, the mixed metal oxides are evaluated catalytically, and the results are correlated to structural findings to establish structure-performance relationships.
What will you learn?
• An introduction to CO2 conversion technologies
• Strategies on how to synthesize and characterize mixed metal oxide catalysts
• Working at the interface of engineering, materials science, and chemistry
• Improve your engineering skill-set and gain practical insight into state-of-the-art chemical/process engineering
Has this sparked your interest? Please feel free to apply or contact us for more information. The scope of the project can be adjusted to the necessary requirements.
Contact:
Alexander Oing
LEE P224
References:
[1] Ye, RP., et al. Nat. Commun. 10, 5698 (2019)
[2] Sternberg, A. & Bardow, A. Energy Environ. Sci. 8, 389–400 (2015)
[3] Kanuri, S. et al. Int. J. Energ. Res. 46, 5503–5522 (2022)
[4] Wang, J. et al. Sci. Adv. 3, e1701290 (2017)
Bachelor / Master thesis / Semester project
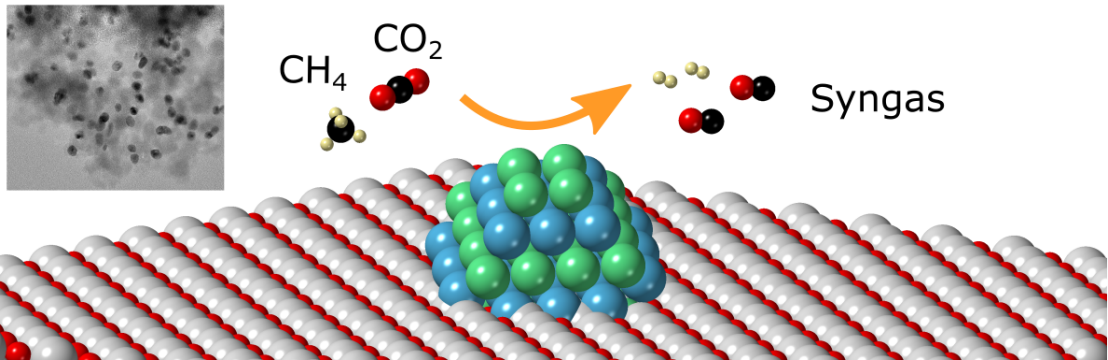
Anthropogenic CO2 is a major concern for society due to its link to global warming [1]. Considering that the energy demand, mainly provided by fossil fuels, is currently increasing at a rate of ~2% per year [2] more sustainable technologies that mitigate or remove CO2 emissions have to be investigated and developed.
The dry reforming of methane (DRM) reacts two major greenhouse gases (CO2 and CH4) to produce syngas (a mixture of CO and H2), with a H2/CO ratio that is favorable for further chemical conversion, e.g. Fischer-Tropsch synthesis. However, side reactions, such as the reverse water-gas shift reaction and methane decomposition, lead to lower selectivities and deactivation of the catalysts which is mainly due to particle growth [3], carbon deposition/coking [4], and/or metal oxidation [5]. There is still a search for catalyst formulations with high stability, regenerability, and activity.
One promising and versatile strategy to yield more active and stable catalysts is the use of bimetallic nanoparticles. The combination of two metals can lead to geometric and/or electronic changes [6], which in turn affect the catalysts’ activity, selectivity, and stability with respect to their monometallic counterparts. However, the synthesis of supported bimetallic nanoparticles with well-defined morphologies, sizes, and crystalline structure remains a challenge.
Our lab is currently interested in developing supported bimetallic nanoparticles for DRM. The goal of this project is to synthesize bimetallic Co-based catalysts via one or two approaches (e.g. impregnation/colloidal), and characterize them in detail (using XRD, ICP-OES, (S)TEM, Raman spectroscopy, etc.). The structure of the obtained catalysts will be correlated with their catalytic performance.
What you will learn
• An introduction to CO2 conversion technologies
• Strategies on how to synthesize monometallic and bimetallic catalysts and how to characterize them
• Working at the interface of engineering, materials science, and chemistry
• Improve your engineering skill-set and gain practical insight into state-of-the-art chemical/process engineering
Has this sparked your interest? Please feel free to apply or contact us for more information. The scope of the project can be adjusted to the necessary requirements.
Contact details
David Niedbalka
CLA H 31
References
[1] IPCC Climate Change 2021: The Physical Science Basis. IPCC: 2021.
[2] Key World Energy Statistics; IEA: Paris: 2020.
[3] Liu, D., et al., Catal. Today 2009, 148 (3-4), 243-250.
[4] Kim, S. M., et al., J. Am. Chem. Soc. 2017, 139 (5), 1937-1949.
[5] Nagaoka, K., et al., Appl. Catal., A 2004, 268 (1-2), 151-158.
[6] Niu, J., et al., ACS Catal. 2021, 11 (4), 2398-2411.
Bachelor thesis / Master thesis / Semester project
Granular materials shape the world. Starting your day with a bowl of cereal and ending it with a walk on the beach, you are surrounded by an uncountable number of solid particles. Major industries like chemical, pharmaceutical, food, building and construction industry handle over 16 billion tons of granular solids each year, making bulk solids handling the world’s largest industrial activity. Despite their importance, granular materials withdraw from an easy physical description as they find themselves located in a state between robust solid bodies and free-flowing liquids. Granular materials possess numerous astonishing phenomena like fluidization, jamming, and segregation, which are challenging physicists and engineers every day.
Our group investigates these phenomena to contribute to a fundamental understanding of granular material behavior. We use cutting-edge technologies such as ultra-fast Magnet Resonance Imaging (MRI) and combine them with numerical simulation techniques like Discrete-Element-Method (DEM) and Lattice-Boltzmann-Method (LBM) to gain valuable insight into the opaque physics behind granular materials. Here is a short selection of our most recent topics:
(i) fluid-solid interactions in three-dimensional fluidized beds,
(ii) diffusion properties and segregation of spherical and non-spherical particles in rotating drums
(iii) jamming in non-Newtonian dense suspensions,
(iv) and hydrodynamics and chemical reactions in packed bed reactors.
Our investigations lead to a closed description of granular materials and pave the way to state of the art industry objectives: process intensification, increased plant and process safety, and readiness for industry 4.0. Get involved with this exciting challenge and support our cutting-edge research on granular materials as part of your thesis, semester project or a student research assistant position. We are constantly looking for students ready to shape the world. So do not hesitate and get in touch with us.
Contact:
Jens Metzger
(Please, for PhD applications use the dedicated link)
What’s in for you?
• Understand the fascinating physics of granular materials
• Get in touch with state-of-the-art experimental techniques and simulation methods
• Enhance your engineering skill-set and get insight into cutting edge research at ETH Zürich
Our laboratory is interested in fundamental studies of electrocatalysts for a range of applications concerning renewable energy conversion and storage, for instance biomass reforming, oxygen reduction, hydrogen and oxygen evolution, towards applications in the fuel cells, electrolyzers, etc. Therefore, we are looking for talented and motivated students who are interested in learning and mastering (1) the synthesis of oxides, carbides, nitrides as bulk solids and nanoparticles; (2) state-of-the-art ex situ and in situ physical characterization techniques; (3) electrochemical characterization of materials.
Currently, the following projects are researched in the group:
(1) Iridium and ruthenium pyrochlore oxides and oxyfluorides investigated to understand the fundamental design principles of oxygen electrocatalysis.
(2) Well-defined noble metal alloys and oxide nanoparticles as electrocatalysts for the application in fuel cells.
(3) Unlocking the potential of two-dimensional carbides and nitrides in electrocatalysis.
(4) Photoelectrocatalytic biomass reforming.
Contact:
Denis Kuznetsov
(Please, for PhD applications use the dedicated link)